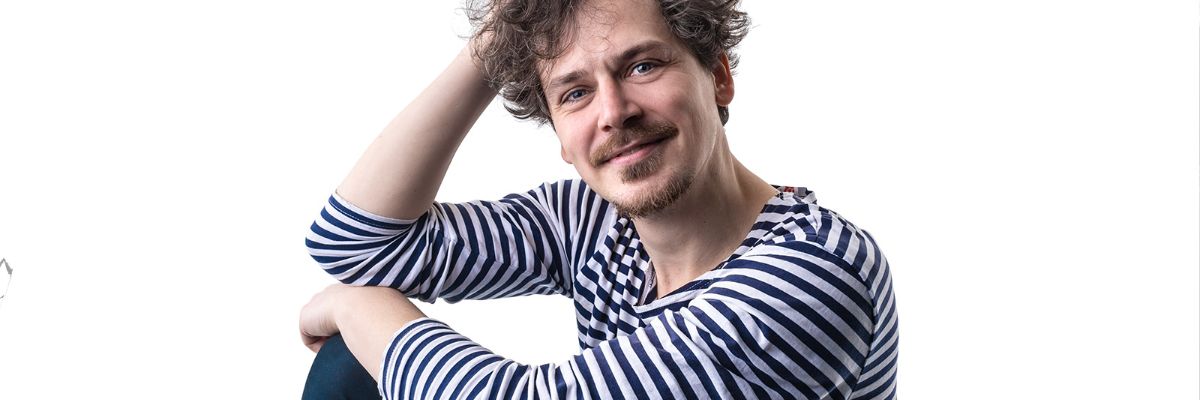
An enthusiast in the world of plant cells
24. 07. 2023
He was deciding between a career as a basketball player and an artist, but eventually, plant cells won out. Surprisingly, the processes occurring in seemingly static flowers are more dynamic and exciting than one might think. And what a feast for the eyes! The interview with the young researcher from the Institute of Experimental Botany of the CAS was published in the CAS Magazine A / Věda a výzkum.
You’d expect a botanist’s office to be teeming with flowers. But I don’t see a single one in yours...
I love plants, but since I’m an experimental botanist, I usually end up killing them in my experiments. I’m interested in the proteins found inside their cells, and the only way to get to them is to crush the flower in liquid nitrogen to a pulp. We then use biochemical methods to get to a small segment that contains the proteins and molecules we want to study. Though some of my colleagues do cultivate an assortment of plants in their offices, it would tempt me too much to experiment.
Are you that interested in what’s inside the flowers?
The inner world of plants is seriously fascinating. You might think that if they are static, their cells would be dull shells without motion. But the opposite is true. Unlike migrating animal cells, they are anchored in place within the cell wall throughout their entire development, but the processes occurring within are considerably faster than those in animal cells.
So while a daisy may not scamper around like a mouse, it is still “busy”.
Yes, it is. Animal cells don’t have a cell wall and can change shape very quickly. Plants can only change shape through growth. And that requires cell division, which involves a lot of movement. You can’t see this with the naked eye, of course, but if you look at the level of molecules and proteins, it’s better than watching an action flick.
What’s so wild about cell division?
While animal and algae cells divide by pinching the mother cell in two, over the course of evolution, terrestrial plants have evolved the exact opposite, centrifugal manner of division. So plants cells divide from the inside out. A cell plate forms in the middle of the cell, which then grows to the edges and separates the two daughter cells from each other. It’s like building a new house in the middle of the cell. And this process is incredibly dynamic.
So the imaginary bricks just go flying, so to speak?
You could say that. In order for the cell to grow, the transport vesicles have to be in action. We can think of this process as a system of freight cars loaded with material that run on a railway line from where the cargo is created to where it’s needed. It’s the same as in our world: the bricks are manufactured in a factory and brought to the construction site where they then get assembled. These freight trains travel in all directions, but when a cell splits, the whole machine has to be redirected to the centre. But in order to do that, they have to receive a signal first. And that’s the job of the lipids, which act here as signalling controls.
They flash a signal and the freight cars set out to the construction site?
Basically, yes. They don’t actually flash or glow, of course, but their physicochemical properties ensure that the freight cars register them. The classic lipid has two legs that don’t like water and a head that loves it. Only lipids with a distinctive head and a large electrostatic charge work as switch towers. But we don’t know yet how they get the idea to signal the cars to kick into high gear and move quickly to the centre. There are still surprisingly large gaps in our knowledge of cell division...
And you’re trying to fill them in.
We’d like to. My team and I are mainly focusing on the cell plate, a fundamental biological structure without which terrestrial plants wouldn’t be able to grow. Because if we prevented it from forming, the cells simply wouldn’t divide. In building the new cell wall, the three main players that enable cells to grow must come together: lipids, polysaccharides, and proteins. And we are trying to unravel and describe exactly how they interact with each other and how it all works in terms of timing and space.
So you’re finding out when and where the different players come into play?
Yes. We use the cell plate as a sort of playground to study the relationships between the various actors. We want to figure out which protein enters the action first, when a polysaccharide starts to appear there, which of the thousands of types of lipids are involved in the division... Going back to the analogy of constructing a house, we are basically figuring out which company is pouring the foundation, who is in charge of the perimeter walls, and who is constructing the roof. We are also interested in finding out why the cell plate looks like a pancake, despite lipids tending to form spherical shapes, and if the pancake is held together by any specific proteins.
Like a sort of scaffolding?
We’re assuming something in the cell works that way. We want to create a detailed 4D map of the cell plate to explain the unique dynamics of plant cell division. This fourth dimension we’re referring to is time. Ideally, there would then be a database from which fellow researchers could download a snapshot of the cell division at a specific point in time. For instance, they could read off which proteins are present at that particular moment and what their function is.
How would that be helpful to them?
If we understand who the key players in the division are and how protein complexes work, we can be careful not to lose them during cultivation. We can also propagate division and increase yields of agricultural crops. If we figure out the basic mechanisms at the cellular level, we may even be able to create more resilient plants.
The kind that you can step on, but they just keep on blooming?
I’d say it’s more about being more resistant to pathogens. When a fungus is trying to infect a tomato plant, for instance, the plant sends the same set of freight cars involved in cell division to the site of the attack. They immediately start to build a new wall, a mechanical barrier, to prevent said attack. Possessing the knowledge of the key players in this process will enable us to create a plant with an even faster defence mechanism. By the way, in moments of such attacks, the things happening in the plants are like scenes taken straight out of Star Wars.
Really?
The plant begins to create new defence proteins. The infected cell moves its entire nucleus to the site of infection so that these new proteins are closer to the pathogen and can better protect the plant. If that doesn’t deter the fungus, the proteins trigger the production of reactive oxygen species that try to puncture the pathogen’s cells. This can be likened to artillery fire. The punctured pathogen cell is unable to survive and the plant then kills it. If worst comes to worst, the tomato plant can even kill off its own cells around the site of infestation, which can cause the fungus to ‘break off’ and prevent the infection from advancing.
Your eyes lit up as you were explaining this. It’s as if you were watching the battle in real time.
I love science fiction, and that’s probably part of the reason why I have a great imagination. But mostly I just find plant cells incredibly fascinating. I really can’t imagine doing anything else. It’s my passion.
You took a rather roundabout path before ending up in the world of plant cells, didn’t you?
That’s true. At first, I wanted to be a basketball player or an artist. Eventually, circumstances led me to studying food science in high school with a focus on food analysis.
That’s what I call a twist!
It is, but in the end, I’m glad it happened. We had to take a lot of microbiology courses, and I found out in the lab how beautiful microorganisms can look under a microscope. They may not have eyes or traditional sensory organs, but they have beautiful colours and shapes. I became fascinated with living systems. But I also enjoyed physical chemistry and making use of maths calculations, so I enrolled in biochemistry at the University of Chemistry and Technology in Prague. However, I became more and more interested in learning about plants. Like what’s behind the fact that each one is shaped differently. Why does a dandelion look different than, say, a hibiscus? Why does one plant have palm-shaped but another fiddle-shaped leaves, for instance?
Why?
They’re different solutions to the same problem. Each plant wants to occupy as much surface area as possible to absorb as much photosynthetically active radiation as possible. But its final shape is defined by its cells. They control everything! And that’s why I wanted to get down to the level of biomolecules and proteins to understand what’s going on. During my PhD, I gravitated more and more towards mathematical modelling, i.e., computer-based work.
How does a person who enjoys dandelions and their shapes get excited about sitting at a computer?
With a computer, you can ‘enjoy the view’, too. When you use a combination of experimental and computational methods to figure out the structure of a protein and visualise it on the screen, it’s a thing of beauty. Even the heterogeneity of proteins, whose structure defines their function, is beautiful in itself. In fact, just by the way they look, we can deduce what they are for and how important they are for a given biological process.
Can you think of an example?
For example, the ATPase enzyme, a protein which produces energy by transporting protons across the membrane, looks like a pump. It has a stator, a rotor... The protons are pumped to one side of the membrane, concentrated there, and the protein allows them to jump to the other side. As they jump, the rotor spins. The mechanical energy is then used for storing it in chemical bonds. From the structure, then, we’re able to guess how it all works. What’s more, we can use molecular dynamics to set the pump in motion on a computer screen – and what a spectacle that can be!
So you spend most of your time at the computer?
When we need to form hypotheses, yes. But we have experimental phases too when we test these hypotheses in the lab. However, since there are only two of us in our team of seven doing the calculations, I work on the computer a lot. My colleagues sometimes tease me about it. Because I create simulations, they always say, “He’s only simulating [feigning].”
You’re laughing, so I’m guessing it doesn’t make you mad...
No, my colleagues and I have a great relationship and I know it’s all just fun. But it’s true that experimental scientists sometimes look down on us “calculators”. They only see pretty pictures, but not their informational value.
Pretty pictures are also constantly popping up on your monitor as we speak. Is your computer working on something all this time?
Yes, it’s running a calculation. That’s one of the advantages of modelling: I give the computer a task and it works independently. Plus, if I set some parameters wrong, the calculation crashes – you find out right away. With traditional experiments, it takes much longer to figure out a technical failure. Every mistake in the lab costs a lot of time. You have to wait several weeks for the biological material to grow, then you have to crush it in liquid nitrogen, and then you can end up pipetting the wrong solution into it, for instance. You can pour it down the drain if that happens.
Which can’t happen on the computer, obviously. Can you explain how you use it, exactly?
Computational chemistry is dependent on programs created by the scientific community. You have to learn to work with them and know the basics of code. For example, I downloaded a program developed by a group of researchers that works basically like a computational microscope, which allows me to examine the molecular level of plants. I also use this kind of virtual cookbook that tells you how different atoms interact with each other.
What kind of recipes can you find in it?
For instance, how hydrogen interacts with carbon in certain molecules. All biomolecules are made up of similar basic units. The cookbook allows us to cook more elaborate recipes, or to describe complex molecules and their functional units. And I can use it to explore any system. In addition, last year revolutionised the prediction of protein structures. Thanks to this we can now look at proteins where we didn’t know what they look like before, download their structure, and use it as input for molecular dynamics. For my work, this is a veritable goldmine.
So you give a computer a task, it goes through all the existing cookbooks and databases, and spits out the result?
Modeling’s been around for about 50 years. So we have a great advantage of being able to draw on what’s already been done. But the process is not as simple as you say. To illustrate, I’ll describe how a particular protein “talks” to the membrane during division. I specify at what temperature and pressure this should happen, how many microseconds of this activity I’m interested in. The computer records the evolution of the system, moves it along at each step, rearranges it... And I use commands to communicate with it. I then apply my biological knowledge of the system and chemical intuition to the results to form hypotheses, which we then test experimentally.
And then it’s time for the pipettes and microscopes?
Yes, we use molecular biology and biochemistry methods, microscopy, mass spectrometry, and others to test our hypotheses. For example, we use calculations to pick out a protein that’s important for division. In the lab, we can then genetically engineer the protein out of the plant or suppress it and see what happens.
What kind of things do you find when you torment a flower like that?
I admit we’re a bit insidious in this, but we need to throw the system off balance, to upend it a bit. It’s the only way we can get nature to reveal its secrets to us. If, for instance, a plant doesn’t grow at all or is stunted in its growth after our intervention, it confirms our hypothesis about the central role of that examined particular protein in cell division. Then we examine what happens when we put it back in – all or just part of it. And in doing so, we can uncover the modus operandi of the protein. This process takes months.
You have to be patient, then...
Like any researcher. In science, you have to be prepared for the fact that ninety percent of experiments fail, eight percent turn out so-so, and a mere two percent of experiments are successful. You have to put your hopes in it and not allow yourself to be discouraged.
This approach is clearly bearing fruit. At the end of last year, you were awarded a JUNIOR STAR grant intended for excellent early-career scientists to research the cell plate. Something like that kicks you into gear, doesn’t it?
Absolutely. Thanks to the grant, we were able to establish the Laboratory of Integrative Structural Biology [at the Institute of Experimental Botany] and create a team of experts in microscopy, computational chemistry, and classical biochemical processes. Because an interdisciplinary approach is essential to our research. In short, we try to take all available methods across disciplines and use them as input for mathematical modelling. Only in this way can we move forward and fill in the blanks in our knowledge of the process of plant division.
You said that without the cell plate, terrestrial plants wouldn’t be able to grow. How is it possible that not everything is yet known about such an important structure?
I have a bit of a half-baked answer: because plant biology is the Cinderella of the biological sciences. It has no medical applications. Structural biology is primarily concerned with proteins that can be applicable to the development of pharmaceuticals. So it’s not as interesting where plants are concerned, which is why there is not as much investment into the research. I remember a colleague from Israel asking me in amazement why we use such complex methods on flowers. And why we don’t try it on animals, so that it would actually be of some use, too (laughter).
That sure must make you feel good about your work...
This approach is still quite widespread. There’s not that much interest in plant cell biology from students, either. Also, scientific papers with this focus are outright ignored by certain biologists. That happened to me at my postdoc in Ghent. We discovered that the so-called TPLATE complex is part of a unique nutrient recycling pathway, and we demonstrated this on plant cells and published the results. A year later, an article was published describing the same pathway in yeast. It totally ignored our paper. But now things are slowly changing – for instance, Chinese plant science is booming and it’s attracting a lot of investment.
You mentioned a fellowship in Belgium. How is the life of a researcher different there versus here?
Our institute in Prague seems more family-like to me. The institute in Ghent is more of a kind of scientific factory where there is a lot of pressure to perform. That’s good, of course, but I have the impression that people enjoy science more here. Maybe there’s also the financial aspect to take into account. Researchers in the Czech Republic usually don’t earn nearly as much as their colleagues in Belgium. And that’s why it’s primarily enthusiasts who remain in science – those, who are really fulfilled by research. Nevertheless, I have fond memories of my time in Ghent.
Is there anything you missed when you returned home?
The fries! They’re just great there. But I definitely don’t miss Belgian beer, although they’re quite proud of it. I prefer Czech beer, especially the one from Pilsen. But back to Ghent. It’s a beautiful, historic city, and full of life at the same time. Some of my colleagues found the frequent rain and monotonous landscape hard to bear. But I don’t mind when it rains, and being from [the Czech town of] Pardubice, I’m used to living on a plateau. Moreover, we formed an excellent international basketball team there and ended up playing several times a week during our lunchbreak.
So you still play basketball?
I don’t think I could do without it. Years ago, when I came to Prague for university, I took a break from it for a while. But during my PhD, I was desperate for exercise, so I took up the sport again. Now I play with my colleagues once or twice a week. I jokingly call it the rainforest league. But we have the whole shebang – official referees, scoreboards, and a game every week.
Speaking of sports, there’s a folding bike next to your desk. Do you ride it home?
Actually, no, I don’t. It was the plan, but it’s just lying around for now, and its current purpose is to foster the illusion that I’m this great athlete in my visitors (laughter). But I’ll start in the spring for sure!
You said that when you were growing up you wanted to be an artist, too. What did you create?
I drew and painted a lot. Everything from portraits to landscapes, so realistic paintings rather than abstract stuff. Until a few years ago, I would often just paint for myself, but I have less and less time for that and, like with the basketball, I’m getting worse and worse at it. I always get some idea of what a drawing should look like. Then I pick up a pencil and, since I’m out of practice, I don’t even come close to my vision. It’s also not as relaxing an activity for me as it should and used to be.
Do you ever regret that you didn’t become a painter or professional basketball player and instead ended up focusing on the “outcast” of biological sciences?
Not at all! On the contrary, I’m very happy, as it’s a challenge for me. I don’t regret not being a basketball player at all. On some days, maybe it’s the art thing that bothers me more. But then I always tell myself that as a mediocre researcher, you can still make a living, but as a mediocre artist... (laughter). Plus, science compensates art for me. When I prepare publications, I have to put together the scientific illustrations and all the materials somehow, which I also see as creation. I can also enjoy all the beautifully coloured protein models every day.
You must be a big fan of colours, right? I can’t help but notice your vivid socks.
I usually get those as gifts. I stopped pairing socks years ago because I think my socks are being swallowed up at home by a blackhole and I could never find the right pair. Then my family started buying me brightly coloured ones – apparently so I wouldn’t look so stupid when I wear unmatched ones. Coloured socks are all the rage now, but I’ve been wearing them for a really long time. When I listen to myself, I sound like a typical hipster – they always say they were wearing something long before it became trendy (laughter).
You look a bit like a hipster, you know...
Thank you. And now I should probably, like a proper hipster, deny that I’m a hipster (laughter).
What’s your favourite way to relax?
Reading. When I’m not at work or with my wife, my ideal downtime is spent with a book. I used to read a lot of science fiction, but now I mostly read biographies, history books, and non-fiction. Or short stories. I’m usually reading ten books at the same time. I should probably finish everything quickly now, though. We’re expecting a baby in May, and I expect that’s going to be a lot (of fun).
Congratulations! Are you looking forward to it?
I am, a lot. But I’m trying to approach it with total humility. I have a lot of respect for parenthood – being a good dad is a big responsibility. I’m a hopeless optimist, though. I’m just going to follow my life motto: don’t be an asshole (laughter)!
Ing. Roman Pleskot, Ph.D. |
He graduated from the University of Chemistry and Technology in Prague, majoring in biochemistry. He continued his doctoral studies at the Faculty of Science at Charles University in Prague and spent a postdoc fellowship working in Pavel Jungwirth’s lab at the Institute of Organic Chemistry and Biochemistry of the CAS and Martin Potocki’s lab at the Institute of Experimental Botany of the CAS. He completed a three-year postdoc fellowship in Ghent, Belgium. Since 2021, he has been the head of the Laboratory of Integrative Structural Biology at the Institute of Experimental Botany of the CAS. Last year, he received a JUNIOR STAR grant for excellent early-career scientists. |
The interview was originally published (in Czech) in the CAS Magazine A / Věda a výzkum:
1/2023 (version for browsing)
1/2023 (version for download)
Prepared by: Radka Římanová, Division of External Relations, CAO of the CAS
Translated by: Tereza Novická, Division of External Relations, CAO of the CAS
Photo: Jana Plavec, Division of External Relations, CAO of the CAS
The text and photographs are released for use under the Creative Commons licence.
Read also
- A trapped state: The pandemic impact on public attitudes, trust, and behavior
- Aerial archaeology: Tracing the footsteps of our ancestors from the sky
- Archaeologists uncover ancient finds along Prague Ring Road
- Our microbiome largely depends on what we eat, says microbiologist Michal Kraus
- The ABCs of writing: Why did its invention mark a turning point for humankind?
- We learn, remember, forget… What can memory actually do? And can we outsmart it?
- New Center for Electron Microscopy in Brno opens its doors to global science
- The hidden lives of waste: What can we learn from waste workers and pickers?
- A unique lab is hidden right beneath Prague’s Vítkov Hill
- Renewables are a strategic investment in European security, scientists say
The Czech Academy of Sciences (the CAS)
The mission of the CAS
The primary mission of the CAS is to conduct research in a broad spectrum of natural, technical and social sciences as well as humanities. This research aims to advance progress of scientific knowledge at the international level, considering, however, the specific needs of the Czech society and the national culture.
President of the CAS
Prof. Eva Zažímalová has started her second term of office in May 2021. She is a respected scientist, and a Professor of Plant Anatomy and Physiology.
She is also a part of GCSA of the EU.