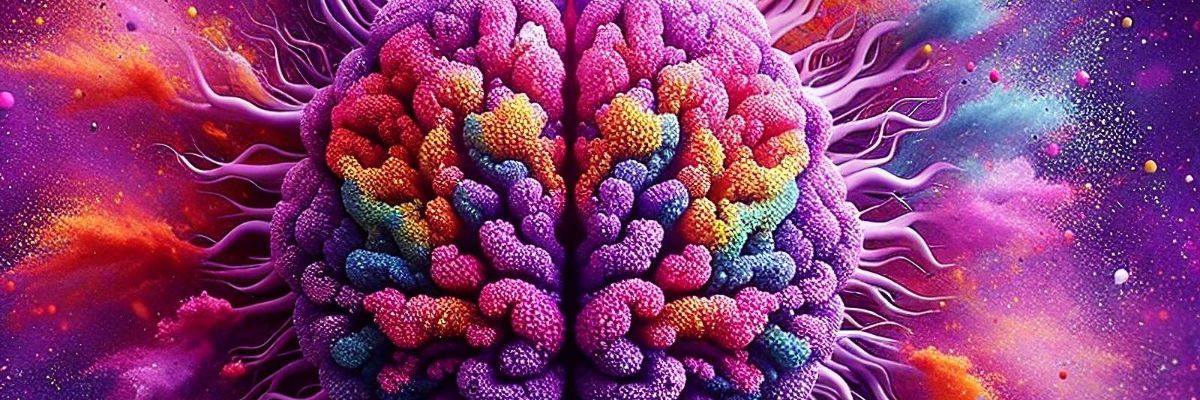
An epileptic seizure doesn’t always strike out of the blue, says Jaroslav Hlinka
18. 12. 2024
A brief blank stare, a sense of déjà vu, loss of consciousness accompanied by convulsions, or even weeks-long confusion – epileptic seizures can manifest in many forms. Most of the time, they occur unexpectedly. But do they really? Computational neuroscientist Jaroslav Hlinka, from the Institute of Computer Science of the Czech Academy of Sciences (CAS), has been modeling the dynamics of epileptic seizures for years. His research was recently featured in the quarterly A / Magazine published by the CAS.
“Goooal!” A loud cheer echoes through the football stadium as euphoric fans in the first row leap up with their arms in the air. Moments later, the second row follows suit, then the third, the fourth… A Mexican wave sweeps through the stands, the crowd chants, and excitement builds.
Something similar happens – albeit figuratively – inside the brain of someone experiencing an epileptic seizure. Instead of fans, it is neurons – nerve cells – that suddenly begin to exhibit high levels of activity. From seemingly nowhere, they begin firing excessively and pull other neurons into the frenzy. A “Mexican wave” of neural activity emerges, with neurons firing wildly, and the overwhelmed brain can no longer function normally. Outwardly, this manifests as the characteristic jerky movements or loss of consciousness associated with seizures.
Jaroslav Hlinka from the Institute of Computer Science of the CAS. (CC)
While the “runaway” neurons put the body through a lot, most patients report that the seizure itself isn’t as distressing as the constant fear of when the next one might strike. Seizures often hit seemingly out of nowhere – completely unpredictably and randomly. However, scientists believe that this unpredictability may not remain a mystery forever.
“Sometimes, seizures are preceded by detectable changes in brain activity. The brain gradually loses stability and resilience, becoming more susceptible to epileptic discharges,” explains Jaroslav Hlinka, a computational neuroscientist who has long studied brain activity, including the modeling of epileptic seizure dynamics, at the Institute of Computer Science of the CAS.
According to him, these changes often exhibit a principle known as critical slowing down – typically occurring when systems lose stability. Critical slowing down can also foreshadow phenomena like climate change, stock market crashes, species extinctions, or even the onset of depression.
The brain in its groove
A piercing ray of sunlight, a gentle breeze, a dog barking, a passerby… Our brain constantly faces countless stimuli to which it must somehow respond. If in good shape, the brain doesn’t get easily disturbed. In this state, it functions well, like a ball resting comfortably in a deep groove, reluctant to leave its stable position.
“If you try to nudge the ball out of the groove, gravity ensures it rolls right back in. Similarly, our brain typically reacts to external stimuli by triggering feedback mechanisms that quickly restore its equilibrium,” Hlinka explains.
But if the brain’s stability is compromised, its responses to minor disturbances become sluggish, and it takes longer to return to its original state. In other words, it exhibits signs of critical slowing down.
Approximately one percent of the population suffers from active epilepsy.
It is in this vulnerable state that the brain becomes vulnerable to epileptic seizures. When the brain is unstable and sensitive, even a seemingly harmless “nudge” from the outside – like a flash of light, an unexpected sound, even a memory – can be enough to trigger a seizure. It’s as if the groove becomes shallow, and the ball, with the slightest push, rolls out of its once-stable resting place.
Individuals with epilepsy typically have no idea that their neurons are “out of form” prior to a seizure. However, technology could potentially provide them with early warnings.
“Electrophysiological methods allow us to measure brain activity with millisecond-level precision. Using sensors, we could monitor the slowing of the brain’s response times to specific stimuli and predict an increased likelihood of a seizure in certain patients, offering them advance warning,” Hlinka explains his research that was published in Nature Communications.
Why just some patients though? Unfortunately, epilepsy is too diverse a condition to be able to apply these findings universally. Experts estimate there are around seventy different types of epilepsy, making it challenging to develop one-size-fits-all predictive tools.
Seizure forecasts?
“In the Central Bohemian Region, we expect the development of cumulus clouds, followed by frequent showers and thunderstorms,” a TV weather presenter announces. Yet viewers remain in the dark about whether it will rain in one particular city or not, where lightning will strike, or at what time – meteorologists simply cannot predict such specifics. The unpredictability of epileptic seizures resembles this scenario.
“We cannot pinpoint that a seizure will happen on a Tuesday at precisely 6:30 PM or predict its exact nature. However, even a general warning about increased seizure risk could be highly practical for patients,” Hlinka emphasizes.
Jaroslav Hlinka focuses on modeling epileptic seizure dynamics. (CC)
Hlinka’s research team, COBRA (short for Complex Networks and Brain Dynamics Group), in tandem with physicians and physiologists, studies brain activity in people with epilepsy – not only immediately preceding seizures, but also over the long term.
“When a cyclist wobbles on their bike, there’s a high probability they’ll soon fall. This ‘wobbling’ before a fall is akin to critical slowing down before an epileptic seizure. What we’re studying is not just the signs of imbalance shortly before an accident, but also why the cyclist is wobbling in the first place, and why at other times they don’t,” explains Hlinka who, in addition to computational neuroscience, studied mathematics and psychology.
Using brain resilience indicators, Hlinka’s team has observed that the risk of seizures fluctuates in people with epilepsy over days, weeks, even months.
“At first glance, seizure occurrence may seem random, as if governed by dice rolls. But long-term measurements show that most patients exhibit at least some degree of regularity,” Hlinka says. This pattern, he notes, might correlate with circadian rhythms – many patients, for instance, experience seizures only at night or early in the morning.
Using data from animal models, Hlinka’s team has also demonstrated that the timing and nature of a previous seizure can influence that of subsequent ones.
The magic of equations
The entire dynamics of an epileptic brain are contained in two key equations. These describe the gradual loss of stability, the transition to a seizure state, its progression and termination, and the recurrence of the whole cycle.
Every fourth to fifth person will experience at least one seizure during their lifetime.
Researchers have translated all this into the language of mathematics, condensing it into just a few symbols. This “translation” has allowed them to simulate the entire system on a computer and develop mathematical models of epileptic dynamics to better understand and predict seizures.
“When a student is asked in school to determine how fast an object falls in a gravitational field, they use the relevant equation. In our case, equations let us calculate how seizures will unfold. Without them, it simply wouldn’t work,” notes Hlinka, who considers solving equations the best form of relaxation.
Combining mathematics and neuroimaging methods to understand how the brain works, which Hlinka and his team focus on, is relatively new both globally and in the Czech Republic. And since this expertise is valuable in many areas of medicine, the Department of Complex Systems at the Institute of Computer Science of the CAS, where the COBRA project is based, has been constantly busy in recent years.
“Neurologists, psychiatrists, and psychologists come to us for help analyzing their data. For instance, we look for differences in brain activity between healthy individuals and those with various diagnoses, develop AI for prognostics, and search for the neural underpinnings of clinical symptoms,” the researcher says.
Hlinka and his colleagues also use mathematics to study the brains of patients with schizophrenia and depression, where statistical analysis and machine learning play significant roles. In contrast, epilepsy is predominantly tackled by a subgroup of “modelers.” According to the researcher, “it seems that, due to the nature of epilepsy, it’s computational neuroscience which has the greatest potential to truly help in this area.”
Electrical sensor implants could potentially prevent seizures
A white plush bunny in a well-known TV commercial from the nineties is happily beating a drum and wiggling to the rhythm – that is, until its batteries run out. Something similar happens during an epileptic seizure: neurons fire so intensely until the brain “runs out of energy” and can no longer continue.
Maps of brain regions and their connections are valuable tools for scientists.
This is why in the vast majority of cases, seizures end on their own. The patient’s brain seems to reset itself after the intense activity of the neurons, returning to a state that resembles normal functioning. However, in people with epilepsy, this capricious organ has a tendency to “recharge” repeatedly, with an ever-growing inclination to “let off steam” by means of another seizure.
How can this vicious cycle be broken? A critical observation helped researchers find a possible answer: they noticed that while certain stimuli trigger seizures, others seem to delay their onset. Hlinka was introduced to this enigma by his colleague Přemysl Jiruška, now the Director of the Department of Physiology at the Second Faculty of Medicine at Charles University. Together, they began searching for an explanation. At the Institute of Computer Science of the CAS, they built a mathematical model of an epileptic brain that behaves like the aforementioned battery-powered bunny. This model gave rise to the suggestion that it might indeed be possible to “postpone” seizures.
“It seems the key lies in the intensity of the stimulus – while a strong stimulus usually acts as the last straw, or rather the match in the powder barrel, that triggers the seizure, smaller perturbations only slightly ‘discharge’ the brain, thereby delaying the onset of the seizure,” Hlinka summarizes the discovery, which the researchers published in Nature Neuroscience.
Their simulations even showed that for some patients, it might be possible to prevent seizures entirely using these small “nudges.” However, this would require determining the appropriate intensity and dosage of the stimuli, as well as figuring out how to deliver these low-intensity “taps” safely to the necessary location.
“All of this could be managed by an electrical sensor implanted in the brain, capable of not only detecting signs of critical slowing down, but also of calculating and sending the optimal impulse to the brain’s control center to ‘discharge’ it just enough to eliminate the need for a seizure,” the researcher proposes.
The taming of the wave
According to Hlinka, several biotechnology companies around the world are already developing such sensors. However, it will still take some time before COBRA’s findings can be applied in practice using these devices.
“Epilepsy is an extensive 'tree' of various types of diseases,” Jaroslav Hlinka says. (CC)
“We need to collect and analyze a significant amount of data from real patients to fine-tune the model. Implanting a sensor into the brain is quite an invasive procedure, so this task won’t be easy. But I hope that within ten years, sensors of this type could become relatively common for people with this diagnosis,” Hlinka says optimistically.
Epilepsy, once known as “the falling sickness” or “the sacred disease,” remains a focus of Hlinka’s research aimed at controlling the “Mexican waves” of neurons. By means of the Brain Dynamics (BRADY) project, supported by the Johannes Amos Comenius Program for cutting-edge research, Hlinka and his team, along with other groups, will explore how computational neuroscience could aid in designing therapies for epilepsy and other brain disorders. “There’s still a lot we have to say on the subject of epilepsy,” the scientist concludes.
*
Ing. Mgr. Jaroslav Hlinka, Ph.D.
Institute of Computer Science of the CAS
Jaroslav Hlinka studied psychology at Charles University and mathematics at the Czech Technical University in Prague. He earned his Ph.D. in computational neuroscience from the University of Nottingham. Since 2009, he has been leading the Department of Complex Systems at the Institute of Computer Science of the CAS. Hlinka’s focus is mainly on modeling epileptic seizure dynamics and researching brain activity during epileptic seizures. He also applies his expertise in mathematics to complex systems such as economic networks and climate models. Last year, he received the Czech Academy of Sciences Award for outstanding research achievements. He also works at the Czech National Institute of Mental Health and lectures at the Czech Technical University in Prague. In 2023, he received the Award of the CAS for outstanding results of great scientific significance.
*
The story first came out (in Czech) in the CAS quarterly A / Magazine.
Written and prepared by: Radka Římanová, External Relations Division, CAO of the CAS
Translated by: Tereza Novická, External Relations Division, CAO of the CAS
Photo: Shutterstock; Jana Plavec, External Relations Division, CAO of the CAS; COBRA archive
The text and photos labeled CC are released for use under a Creative Commons license.
Read also
- Radomír Pánek nominated for the next President of the CAS
- Four CAS projects get ERC Consolidator Grants, each to receive two million euros
- Groundbreaking study maps brain’s recovery process after stroke
- A / Magazine: light, the dawn of quantum computing, and no man’s lands
- Of Mice and Men: Researchers reveal link between human and mouse migration paths
- Czech and Saxon Academies of Sciences to strengthen cooperation on key issues
- Laser micromachining: inspired by sharks
- CAS researchers received the 2024 Praemium Academiae and Lumina Quaeruntur
- Light – Illuminating the invisible
- Interview | Julius Lukeš: The enfant terrible of Czech science
The Czech Academy of Sciences (the CAS)
The mission of the CAS
The primary mission of the CAS is to conduct research in a broad spectrum of natural, technical and social sciences as well as humanities. This research aims to advance progress of scientific knowledge at the international level, considering, however, the specific needs of the Czech society and the national culture.
President of the CAS
Prof. Eva Zažímalová has started her second term of office in May 2021. She is a respected scientist, and a Professor of Plant Anatomy and Physiology.
She is also a part of GCSA of the EU.