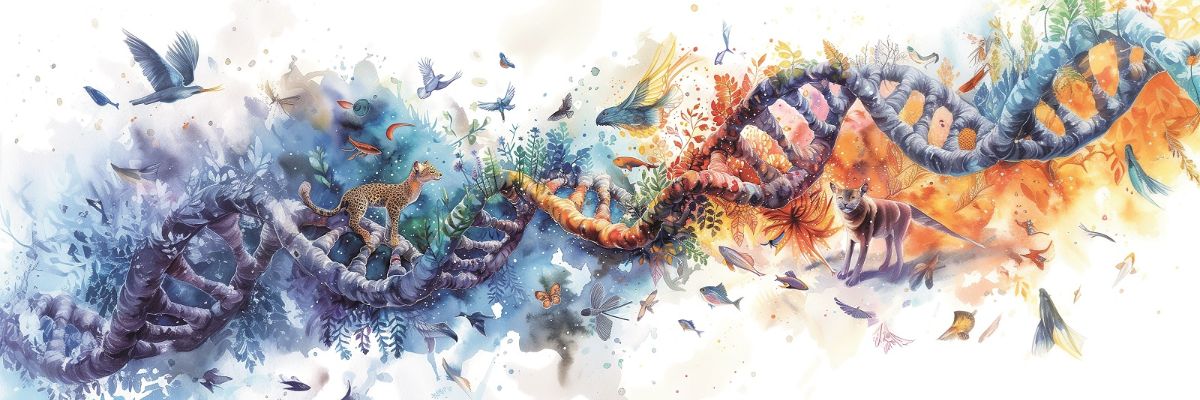
Evolution, alive and well. What lies at the heart of biodiversity?
18. 04. 2024
It’s been 165 years since the first edition of Darwin’s groundbreaking book On the Origin of Species came out. Despite advancements of the most modern research methods, the fundamental questions remain the same. How do new species emerge? And what is ‘the point’ of biodiversity? Read the feature article in A / Magazine to learn about the projects being worked on by Miloš Macholán from the Institute of Animal Physiology and Genetics of the CAS as well as Jaroslav Piálek and Josef Bryja from the Institute of Vertebrate Biology of the CAS. You will learn that a mouse hybrid zone runs through the Czech Republic, why Ethiopia is an open-air laboratory, and why the evolutionary tree of the Muridae family had to be redrawn.
From a young age, Charles Darwin had always been fascinated by nature and the world around him, burning with a desire for knowledge and discoveries. So when the opportunity arose for him to join the second expedition of HMS Beagle at the age of just twenty-two in 1831, he didn’t hesitate for long. The biggest challenge was to convince his father, who initially objected to his son’s journey, considering it a waste of time. With the considerable help of his uncle, the young man managed to convince his father to change his mind – and it’s a good thing he did. Nearly five years of traveling through little-explored corners of South America, southern Africa, Australia, and New Zealand completely changed not only his own life, but also significantly influenced the entire scientific field to which he then decided to devote himself.
Darwin spent two-thirds of the expedition studying the diverse nature on dry land. In Argentina, he studied the fossils of large mammals, while on the Galapagos Islands, he observed various species of finches and giant tortoises, collecting a number of specimens to bring back to Great Britain for further study. While examining the regional biological diversity, he asked questions about how new species emerge in different places and times and why it is that some of them become extinct. He systematically recorded and contemplated his findings, while also meticulously studying the conclusions of other naturalists of his time. In 1859, he then published the book On the Origin of Species by Means of Natural Selection, or the Preservation of Favoured Races in the Struggle for Life, in which he presented extensive evidence of the gradual evolution of organisms and introduced the concept of natural selection as the key mechanism of evolution.
Evolution reimagined: a revolutionary concept
“Until recently the great majority of naturalists believed that species were immutable productions, and had been separately created. […] Some few naturalists, on the other hand, have believed that species undergo modification, and that the existing forms of life are the descendants by true generation of pre-existing forms,” Darwin writes in the preface to his book.
Although we commonly perceive him today as the father of evolutionary theory, Darwin was not the first to come up with this hypothesis. Darwin himself refers to the French thinker Jean Baptiste Lamarck in his book, who mentioned the development of species as early as the beginning of the 19th century. Even Charles Darwin’s own grandfather, Erasmus Darwin, formulated evolutionary ideas in his work Zoonomia at the end of the 18th century.
“Most people may be surprised to learn that the first evolutionists also included the famous poet and playwright, Johann Wolfgang Goethe. Probably only a few experts in the Czech National Revival period will know that one of our most distinguished naturalists of that time, Jan Svatopluk Presl, wrote about evolution in his General Botany from 1846,” says evolutionary biologist Miloš Macholán from the Institute of Animal Physiology and Genetics of the CAS.
However, the fact that several experts were dedicated to similar ideas at the same time does in no way diminish the significance of Charles Darwin. He is regarded as the founder of modern evolutionary theory, and rightly so, because he was able to describe evolution in a comprehensive manner with his systematic approach and understandable language, placing it in the necessary context. Most importantly, he connected evolution with the theory of natural selection, which helped explain why and how species evolve.
The Moravian lineage
The most significant challenge that Darwinian evolution faced in its time was the lack of a corresponding theory of biological inheritance that could explain the preservation of intergenerational variations, supposed to be connected to natural selection. The missing link was Mendelian genetics. It may be hard to believe, but despite the fact that the Augustinian friar Gregor Johann Mendel published his discovery of the rules of heredity in 1866, Darwin never learned about them – just like most naturalists of that time, in fact.
It wasn’t until 1900 that several scientists rediscovered and independently verified the Mendelian laws of inheritance. Two to three decades later, different theorists then connected the mathematical principles of genetics with Darwin’s theory of natural selection. “In my opinion, the integration of Darwin’s theory of evolution and Mendel’s theory of heredity represents one of the most significant milestones in the history of evolutionary biology. Neo-Darwinism marked a major breakthrough,” Macholán explains.
Prof. RNDr. Miloš Macholán, CSc.
Macholán studied general biology at the Faculty of Science at the University of J. E. Purkyně (now Masaryk University) in Brno. He now leads the Laboratory of Mammalian Evolutionary Genetics at the Institute of Animal Physiology and Genetics of the CAS. He also teaches at Masaryk University. In 2002, he received the Otto Wichterle Award. He is a co-editor of the book Evolution of the House Mouse (2012, Cambridge University Press). In 2022, he received the Academia Publishing Award for co-authoring the book Systém a fylogeneze savců (together with Jan Zima). His research focuses on genetic and phenotypic variability and systematics of small mammals, the evolution of the genus Mus, hybridization, and speciation of the house mouse. |
Evolutionary laboratories
Evolutionary theory can be approached from many different angles. We can discuss its history and development in a broader scope, or even acknowledge the passionate (primarily American) debate between evolutionists (who advocate a scientific stance) and creationists (who promote a literal interpretation of the Bible). Or, we can keep our feet on the ground and focus more on specific scientific projects that further our understanding of the origin and evolution of animal species.
For this article, we chose the latter path. For instance, we will open the “window on the evolutionary process,” as hybrid zones are sometimes called. These are geographical areas in which two genetically distinct populations meet, interbreed, and produce hybrid offspring. Can a new species emerge there? Why can’t individual species interbreed? Which genes are responsible for speciation, i.e., the formation of new species?
We will also metaphorically visit an “open-air evolutionary laboratory” – areas of intense biological diversity development, perhaps due to exceptional climatic conditions. One such place is the Ethiopian Highlands, which has been confirmed by recent research from Czech scientists as one of the key cradles of biodiversity in Africa.
How does a species emerge?
That a diversity of animal and plant species exist is evident. Just take a look at the bird feeder in the winter months – you’d probably manage to distinguish a sparrow from a tit or a magpie. Similarly, nobody would likely mistake an oak tree for a larch. In nature, different species are simply able to coexist within the same environment. But why, exactly? How is it possible that over time, species like sparrows and tits don’t interbreed and form a new species? Conversely, why are various dog breeds commonly interbred despite not looking like each other at all?
Something incompatible with other species must be present within the genetic makeup of every species. Evolutionary biologists studying speciation are interested in what’s at the core of this genetic barrier, asking questions like: How many genes cause reproductive isolation and how big is their impact? Do they interact? Is their evolution driven by natural selection, or are they products of random processes?
Seeking answers in animals like tits and sparrows would be too complex because at the genetic level, their differences are relatively large – a lot of time has passed since their evolutionary paths diverged from a common ancestor. That’s why it’s necessary to focus on species that are in the process of formation – those between which the reproductive barrier is not insurmountable.
There are basically two possible strategies to study reproductive barriers. The first involves experimental hybridization, where scientists cross individuals from different species in the lab under clearly defined conditions. The other strategy utilizes hybrid zones, geographic regions in nature.
Using the first method, Chung-I Wu and his colleagues from the University of Chicago discovered a speciation gene in vinegar flies in 1993, belonging to the genus Drosophila, which belong to the most conveniently utilized lab animals. The gene bears the poetic name “Odysseus” (abbreviated as OdsH) after the legendary Greek hero. “Just like Odysseus, who, with his comrades, cunningly managed to infiltrate impregnable Troy hidden in the belly of a wooden horse, this gene, once it finds itself inside a foreign genome, causes male sterility, that is, essentially their genetic death, from an evolutionary standpoint,” Macholán explains.
Next stop, the hybrid zone
However, what has been discovered in fruit flies may not necessarily apply to other organisms. For instance, in mice, which are also used as common laboratory animals, dozens or even hundreds of genes with various effects can be involved in reducing the fitness of mouse hybrids.
Mice, however, have a different advantage – to study their hybridization, it is possible to make use of their hybrid zone – a unique natural laboratory where two subspecies of the house mouse meet. Part of this hybrid zone passes through the western tip of the Czech Republic.
“The ancestor of the house mouse emerged approximately half a million years ago. Mouse populations then diverged somewhere in the plains of present-day Iran and gradually spread throughout the world. One group migrated north, above the Caucasus, and the other headed south along the coast of the Mediterranean Sea, either by themselves or with humans on ships,” explains Jaroslav Piálek from the Institute of Vertebrate Biology of the CAS.
Mice that came via the northern route across the continent gave rise to the subspecies Mus musculus musculus. They colonized Eastern and Central Europe, northern Asia, and the Far East. So, if you find a mouse in your pantry at home, it’s likely to be this particular subspecies. However, if you have a cottage somewhere near Cheb, your mouse probably belongs to the western subspecies Mus musculus domesticus. This subspecies evolved from mice that arrived in Europe via the southern route through the Middle East and the Mediterranean.
During the long journey, the genetic makeup of the mouse populations altered, although (still) not enough to diversify them into distinct species. Both subspecies – referred to simply as western and eastern – then encountered each other in Central Europe. They interbred and produced hybrid offspring, forming a belt of hybrid populations, which is over 2,500 kilometers long but only about 10 kilometers wide, stretching from Scandinavia through western Czech Republic and Bavaria to the Balkans and the Black Sea.
A map of the mouse hybrid zone that runs partially through the Czech Republic.
“In the hybrid zone, genetic traits that are typical for each subspecies change, and we can use this reality-cum-model to study speciation,” Piálek adds. To conduct the research, mice from both subspecies need to be captured, their DNA samples taken and subjected to detailed analysis. Additionally, for over twenty years, the Institute of Vertebrate Biology of the CAS in Studenec has been building special colonies that inhabit not the typical lab mouse strains, but rather inbred lines derived from wild populations of both subspecies and other mouse species (referred to as WDS – wild-derived strains). Currently, the Studenec breeding facility houses over two thousand individual mice from ninety different strains.
The advantage of wild mice, including those bred in captivity, is that they have a greater genetic diversity compared to selectively bred lab mice (which usually originate solely from the western subspecies of the house mouse). Some time ago, after long-term efforts, Forejt’s team at the Institute of Molecular Genetics of the CAS identified one of the main genes of mouse hybrid sterility (the first speciation gene described in mammals), Prdm9. However, the discovery pertained to hybrids of wild mice and lab mice, while the mechanism of sterility is more complex in natural hybrid zones. There are records of over four hundred variants of the Prdm9 gene in the house mouse alone.
To learn more, researchers cross males and females of each strain in the Studenec breeding facility and observe which types of crosses yield infertile offspring. They are able to determine from molecular analysis which combination of alleles (variants) of a given gene each strain carries, and from there, they deduce which combinations result in sterility.
“It turns out that there are more negative combinations of Prdm9 gene variants than those previously described. Moreover, various mechanisms can lead to sterility, and it can be encoded by different genes on the X chromosome. In other words, sterility must have evolved multiple times in the mouse during its evolution,” Piálek adds.
graduated biologist Jaroslav Piálek, CSc.
Jaroslav Piálek graduated from the Faculty of Science at the Jan Evangelista Purkyně University (now Masaryk University) in Brno. He completed his doctoral studies at the former Czechoslovak Academy of Sciences (Institute of Systematic and Ecological Biology) in Brno. In 1994–1995, he conducted postdoctoral research at the University of Edinburgh. Together with Jan Zima, Piálek established the Institute of Vertebrate Biology of the Czech Academy of Sciences in Studenec, which he also led from 1998 to 2010. He oversees the breeding facility for mouse strains derived from animals originally captured in the wild (wild-derived strains, WDS). He is one of the editors of the book Evolution of the House Mouse (2012, Cambridge University Press). |
The rivalry of genes
Charles Darwin introduced a sophisticated concept of natural selection, which drives evolution. According to the naturalist, individuals that better adapt to changing environments survive and thrive in subsequent generations, while those with harmful or impractical deviations are doomed to extinction. Darwin illustrated this hypothesis using numerous obvious examples from nature. However, today we know that selection and conflicts occur at the molecular level, too. Since the 1960s, evolutionary theory has been working with the term “selfish gene,” popularized by Richard Dawkins in the late 1970s.
According to the selfish gene theory, the main driver of natural selection is not the individual or the group, but the gene. Individual alleles compete to be represented in future generations, and organisms as a whole are merely their “vehicles,” which they have constructed to more effectively pass on their copies.
“The selfish gene theory introduced a new, gene-centered view into evolutionary theory. Such changes in perspective are usually stimulating and inspiring for further discussions, which holds true in this case,” Macholán comments. “It’s true that today we know of many cases where a particular gene or group of genes behaves as if they’re acting independently of the consequences for their carriers,” he adds.
One interesting example of conflict at the genomic level, leading to an “arms race” between genes, was discovered by Macholán and his colleagues in the mouse hybrid zone. It is known that offspring resulting from hybrid crosses tend to be less viable and fertile. According to Haldane’s rule, these problems specific to hybrids mainly affect males (or individuals of the heterogametic sex, which in mammals are males carrying the sex chromosomes X and Y).
If this rule were indeed true, over time, natural selection would disproportionately impact hybrid males to a greater degree compared to female mice. In other words, the Y chromosome (which only males have) should not pass through the hybrid zone.
“Some results, for example from Denmark, suggested this, but we discovered extensive areas where the Y chromosome of the eastern subspecies massively infiltrates the territory of the western subspecies. I think this surprised many foreign colleagues; it’s truly extraordinary,” Macholán says.
“We have proven that this phenomenon is related to the ‘war’ between genes on the X and Y sex chromosomes. Because females have two X chromosomes and only males have one Y chromosome (XY), the two chromosome pairs have different evolutionary interests, which manifest as either an attempt to skew the sex ratio in favor of females or, conversely, males,” the evolutionary biologist adds.
Despite the evident struggle between “male” and “female” chromosomes, mouse populations remain stable in the long term. Thus, this hypothetical “Maidens’ War” does not decimate all male mice. That’s because eventually, the mechanism of natural selection ends up restoring the sex ratio back to normal, i.e., roughly one to one.
Ethiopia – an open-air laboratory
The belt of the house mouse hybrid zone that partially runs through the Czech Republic offers a crucial “window into the story of evolution.” Another interesting region – albeit for slightly different reasons – is the Ethiopian Highlands. It also provides us with the opportunity to glimpse into the process of species formation through natural selection.
Although it is farther from their homeland than the mouse hybrid zone, Ethiopia has become one of the key research destinations for Czech biologists. “It was a bit of a coincidence, as many things in science end up being. We had a project to research the mechanisms of biological diversity formation in Eastern Africa spanning from Kenya to Mozambique. We made a short, somewhat spontaneous expedition to Ethiopia and were captivated by how different it was from the rest of the region,” recalls Josef Bryja from the Institute of Vertebrate Biology of the CAS.
Ethiopia, with its unique landscape, is actually quite atypical for Africa as we commonly envision it. Out of low-lying savannas, deserts, and semi-deserts, where the lowest point lies 115 meters below sea level, emerges the monumental massif of the highlands. Its highest peaks reach impressive heights – Mount Tullu Dimtu in the Bale Mountains measures 4,377 meters, and Ras Dashen in the Simien Mountains even reaches 4,550 meters. The entire massif is split in two by the Great Rift Valley, which is filled with lakes surrounded by an arid savannah. The region is also carved by other deep valleys with large rivers, such as the Blue Nile. The presence of both high mountains and deep rifts, along with a relatively high degree of geographical isolation from other African mountains, has given rise to a unique natural laboratory for various evolutionary experiments.
Since 2012, Bryja’s team has visited East Africa repeatedly. They have established relationships with local experts, and already a second Ethiopian PhD student is now working at the institute’s facility in Studenec, while Czech biology students from Masaryk University have the opportunity to spend a semester at an Ethiopian university.
The Ethiopian terrain is captivating to researchers due to its relatively unexplored nature. The numbers of officially described species have long indicated that the greatest biodiversity in Africa was to be found in former British colonies – like in the mountains of Uganda or Kenya. The reason for this is rather prosaic – simply that the taxonomists of the British colonies had actively described species diversity in their colonies at the beginning of the 20th century. Evolutionary studies from other parts of Africa, including the unique Ethiopian Highlands, which were never colonized by Europeans, were missing. Moreover, until the 1980s, the local mountain peaks were essentially inaccessible to researchers, as there were no roads leading to them. This changed only recently.
“There are very steep hills, but they can be conquered relatively easily nowadays. In the Bale Mountains, you can drive from about 800 meters to 4,500 meters in a few hours, passing from savanna through mountain tropical forests and gradually decreasing heathlands until you reach the tundra. You soon find yourself in a different world altogether; up there, you feel like you’re somewhere in Norway,” Bryja says.
Each of the mentioned ecosystems is inhabited by representatives of entirely different fauna. Over the course of evolution, the animals adapted to the steep gradient of ecological conditions (contingent on altitude), and very strong selective pressure led to the evolution of entirely original organisms adapted to specific conditions. Such plants and animals are called endemics, meaning they are found only in one particular region of the world.
An example of an animal which can only be found in the Ethiopian Highlands is the Ethiopian wolf (Canis simensis), also known as the red jackal.
In Ethiopian mammals, endemism reaches more than 10 percent – among the most well-known examples are the Ethiopian wolf, mountain nyala, and gelada baboon. For shorter-range groups, the level of evolutionary uniqueness is even greater. For instance, more than 40 percent of Ethiopian rodent species occur only in various ecosystems of the Ethiopian Highlands. Certain species have inhabited the local isolated highlands in almost unchanged forms for millions of years (we call them paleoendemics). At the same time, many “new” species also live there, meaning those that have diversified due to changing conditions (from an evolutionary perspective) only recently.
Bryja’s team focuses primarily on the research of small mammals in sub-Saharan Africa, such as insectivores, elephant shrews, and rodents. It is precisely these groups in which gradual morphological and genetic evolutionary changes can be explored relatively well. Detailed analyses have already borne a number of surprises.
A successful mouse odyssey
One of the first unexpected discoveries came shortly after the initial journey to Ethiopia. Bryja and his colleagues set traps every day during their expedition, aiming to capture small mammals for genetic analysis. Luck wasn’t always on their side; among the hundreds of traps they set every afternoon and checked the morning after, they sometimes only caught one or two animals. However, their luck turned around once they climbed to an elevation of over 3,000 meters. In the alpine tundra of the Arsi Mountains, they trapped a mouse with a relatively short tail and a black stripe on its back. The scientists suspected which species it could be, but weren’t sure. Based on its appearance, they estimated it to be an individual of the Muriculus genus – the stripe-backed mouse (Muriculus imberbis).
“It is a very rare animal. Until then, it was only known from a few old museum exhibits and was considered extinct. No one really knew where it belonged within the evolutionary tree, so it was classified into a separate rodent genus,” Bryja explains. “By sheer chance, we were able to capture a live specimen on our very first expedition in 2012. I was very curious about the outcome of the genetic analysis,” he adds.
The evolutionary tree of the Muridae family is enormous, comprising over 150 genera and 800 species. After the Czech discovery, it had to be redrawn, because it turned out that the mysterious Muriculus actually belongs to a different branch. Rather than forming a separate genus, it is a species of the Mus genus (just like, for instance, the house mouse).
As previously noted, members of the Mus genus dispersed globally from Asia. In Africa, they then formed their own group, which further diversified. “We reconstructed the phylogeny of the entire genus in Africa and found that the first radiation, or species diversification, occurred precisely in the territory of present-day Ethiopia,” Bryja notes. The ancestor of the present-day stripe-backed mouse diverged from most other African mice relatively early in the beginning of the Pliocene (about four to five million years ago) and, along with several other species, remained a so-called paleoendemic in the East African mountains.
About three million years ago, a number of mice from this genus descended from the mountains to the savanna and adapted to the new living conditions. Their bodies decreased in size by roughly three times, allowing them to colonize extensive savanna areas across the entire continent. While mountain mice are only slightly smaller than, for instance, classic lab mice, savanna mice in Africa, weighing 3–5 grams, are among the smallest mammals in the world (as a result, the entire subgenus gained the apt scientific name Nannomys).
Prof. Mgr. et Mgr. Josef Bryja, Ph.D.
Josef Bryja studied Systematic Biology and Ecology (1992–1997) and Molecular Biology and Genetics (1993–1998) at the Faculty of Science, Masaryk University. In 2008, he received the Otto Wichterle Award and completed fellowships in France and Portugal. Since 2010, he has been a principal researcher and head of the external research facility of the Institute of Vertebrate Biology at the CAS in Studenec. He teaches at Masaryk University in Brno. Bryja has participated in numerous international expeditions (Senegal, Ethiopia, Kenya, Uganda, Tanzania, and Mozambique). His research focuses on the taxonomy and phylogenetics of small mammals and their pathogens and the molecular ecology of vertebrates, particularly rodents (but also other groups, such as fish). |
A cradle of diversity
Scientists reached similar findings regarding another rodent species – the big-headed African mole rat (Tachyoryctes macrocephalus). “Globally, it can be found only in the Afroalpine tundra of the Bale Mountains in Ethiopia, at elevations above three thousand meters. It’s truly a peculiar creature,” Bryja notes. The big-headed African mole rat is a giant among rodents (as evidenced by another variant of its name – the giant root-rat). It weighs up to one kilogram and has large protruding teeth and eyes on top of its head, serving as a periscope to survey its surroundings. It inhabits the relatively safe underground burrows but ventures above ground for food, where it becomes the favoured prey for the Ethiopian wolf (the most endangered canid in the world).
All other mole rats found elsewhere in Africa, such as in Uganda or Kenya, are half or one-third the size and live much more covertly, hardly ever leaving their underground burrows. These smaller animals have been classified by British taxonomists into eleven different species.
“Our genomic analysis, however, showed that everything is completely different. The evolutionary diversity of mole rats [root-rats] in Ethiopia is enormous, and the giant root-rat in Bale is just one of the many internal branches on their phylogenetic tree,” Bryja explains. “Due to the extreme environment of the alpine tundra, its body grew in size several times over, and its eyes moved to the top of its head. Conversely, all non-Ethiopian mole rats are the result of a single colonization originating from the Ethiopian mountains; genetically, they are almost identical. With a single study, we essentially invalidated eleven distinct species,” the biologist says with a grin.
The remarkable big-headed African mole rat (Tachyoryctes macrocephalus) inhabits one single place in the world – the Afroalpine moorlands found in the Bale Mountains of Ethiopia.
That the Ethiopian Highlands are a crucial cradle of African biodiversity is further evidenced by the example of the white-toothed shrew – small representatives of the order Eulipotyphla. White-toothed shrews, with their more than 220 species, are the most species-rich genus of mammals in the world. Researchers gathered genetic data from 511 samples spanning the entire East African mountain range – the most extensive dataset of white-toothed shrews ever collected.
“Based on genomic analyses, we identified six distinct evolutionary groups amid the white-toothed shrews. Five of them were found in the Ethiopian Highlands, indicating that, similar to the mole rats, this is where their initial diversification occurred. From here, they subsequently spread to other parts of Africa during two migration waves,” Bryja explains.
The analyses also identified several new species in the Ethiopian mountains, Kenya, and Uganda. Some of them weigh a mere three grams and measure no more than five centimeters. Similar to the aforementioned savannah mice, these shrews are among the smallest mammals in the world.
A mouse-sized elephant
Another example of extraordinary genetic diversity in East Africa is provided by the elephant shrew. At first glance, an untrained eye might classify an elephant shrew somewhere between a mouse (rodent) and a mole (insectivore). They have elongated slender snouts and scaly tails resembling those of rats. However, molecular-genetic analyses conducted over two decades ago revealed that elephant shrews belong to the ancient group Afrotheria. It’s almost unbelievable that such a disparate group as Afrotheria includes elephant shrews and golden moles alongside aardvarks, much larger elephants, and even sea cows.
Afrotheria – as the name suggests – consists of mammals of African origin. Evolution-wise, their ancestors diverged from other placental mammals roughly 100 to 80 million years ago, and after the fragmentation of the supercontinent Gondwana, they continued to evolve for tens of millions of years in African isolation. The oldest fossil evidence of these animals dates back to the early Tertiary period. Although the relationship between elephant shrews, elephants, and sea cows may not be apparent at first glance, genetics provides clear evidence.
External likeness may not necessarily indicate evolutionary relatedness. Elephant shrews and elephants gradually evolved into differently looking animals because both species adapted to different environmental conditions. The elephant shrew resembles a mouse or a shrew precisely because it shared a similar habitat with them. This phenomenon is called convergent evolution.
The black and rufous elephant shrew (Rhynchocyon petersi) is a species of elephant shrew. Previously, it was thought to be related to insectivores, rabbits, or even ungulates. Today, it is classified as a separate order within Afrotheria – a broad group of orders of African origin, which includes elephants, hyraxes, and aardvarks.
Although elephant shrews are found across much of Africa, knowledge about them is incomplete. Most studies have focused on individuals from South Africa and neighboring countries, while the eastern part of the continent remained on the periphery of interest. Over the past fifteen years, the Czech research team has been able to collect new, extensive genetic material of the elephant shrew from East Africa – from Ethiopia, Kenya, Tanzania, Zambia, Malawi, Mozambique, and unique samples from Angola. The results once again led to the rewriting of taxonomic tables.
Genetic analysis, for example, revealed a close relatedness between the rufous elephant shrew (Elephantulus rufescens) and the Somali elephant shrew (Elephantulus revoilii). This result may please shrew experts, but it doesn’t mean much to the average layperson. However, an interesting story is attached to the discovery. The Somali elephant shrew was long considered extinct, the last living individual sighted some fifty years ago. It wasn’t until February 2019 that a team of American researchers happened upon a population of this animal in the arid semi-deserts of Djibouti. Genetics then showed that they represented a highly divergent branch of elephant shrews, leading biologists to create a new genus – Galegeeska.
Although the genome sequences of the much more common rufous elephant shrew were available in the public GenBank database, American scientists failed to uncover their relatedness, while Czech colleagues found in their analyses that these two species are genetically very closely related. How is this possible? “Human error, most likely. Specifically, a misidentification of the previously used material. The sequenced fragments of several genes, which were labeled as the rufous elephant shrew in the gene bank twenty years ago, actually belonged to the short-snouted elephant shrew of the genus Elephantulus,” Bryja explains.
This anecdote illustrates the importance of fieldwork and collecting new samples, not just relying on previously described specimens in gene banks. In 2024, Bryja and his team plan to fly back to Africa, specifically to the Central African Republic. This poorly explored region has yielded almost no data so far. The goal of the planned project is to fill in the gaps in the map of the continent’s biological diversity and to describe as realistically as possible which areas are the richest in evolutionary diversity.
The second reason driving Czech biologists to return to Africa, including to less safe countries, is concerns about the future of local biodiversity. The rapidly growing human population in sub-Saharan Africa is exerting unprecedented pressure on natural ecosystems, threatening to wipe out a large part of the natural diversity before scientists will have time to describe and explore it. That’s why it’s important to know where the most biologically significant regions are located and to focus conservation efforts there.
“The impact of human activity is currently leading to a rapid acceleration of animal species extinction. The emergence of new species occurs through evolutionary development over tens to hundreds of thousands of years, whereas irreversible changes in ecosystems associated with extinction are happening within mere decades,” Bryja adds.
For biologists, fieldwork is a must. The photo above is from an expedition of Josef Bryja's team to Uganda in 2021.
A journey through time
Where the current situation will evolve in the future remains uncertain. The evolution of organisms is an incredibly long-term process, which is difficult to comprehend from the perspective of a short human lifespan. But what if we had the hypothetical opportunity to travel back in time? Which stage of Earth’s history would we choose to explore, which enigmas of its evolution would we like to unravel?
“It would certainly be thrilling to have the chance to study, for instance, the first replicating RNA molecules from four billion years ago, while paleontologists would undoubtedly seize the opportunity to observe the biology and ecology of long-extinct organisms, and so on,” Macholán speculates. “However, from an evolutionary perspective, such time traveling wouldn’t actually help us all that much. Imagine if we were to travel back several million years. Sure, we’d see a vastly different world, which would be interesting in itself. However, that world would appear as static to us as the present one. With precious few exceptions, evolution occurs over a vast period of time.”
The full article can be found (in Czech) in the A / Magazine quarterly published by the Czech Academy of Sciences:
1/2024 (version for browsing)
1/2024 (version for download)
Written and prepared by: Leona Matušková, External Relations Division, CAO of the CAS
Translated by: Tereza Novická, External Relations Division, CAO of the CAS
Photo: Shutterstock; Josef Bryja’s archive; Jana Plavec, External Relations Division, CAO of the CAS
The text and photos labelled CC are released for use under the Creative Commons licence.
Read also
- Fossilized memories: A glimpse into Prague’s Jewish cemeteries
- Newly discovered alga could help unlock the secrets of plant evolution
- How to reinforce the human body with biodegradable metals
- Czech scientists contribute to developing eco-friendly, affordable solar cells
- An epileptic seizure doesn’t always strike out of the blue, says Jaroslav Hlinka
- Radomír Pánek nominated for the next President of the CAS
- Four CAS projects get ERC Consolidator Grants, each to receive two million euros
- Groundbreaking study maps brain’s recovery process after stroke
- A / Magazine: light, the dawn of quantum computing, and no man’s lands
- Of Mice and Men: Researchers reveal link between human and mouse migration paths
The Czech Academy of Sciences (the CAS)
The mission of the CAS
The primary mission of the CAS is to conduct research in a broad spectrum of natural, technical and social sciences as well as humanities. This research aims to advance progress of scientific knowledge at the international level, considering, however, the specific needs of the Czech society and the national culture.
President of the CAS
Prof. Eva Zažímalová has started her second term of office in May 2021. She is a respected scientist, and a Professor of Plant Anatomy and Physiology.
She is also a part of GCSA of the EU.