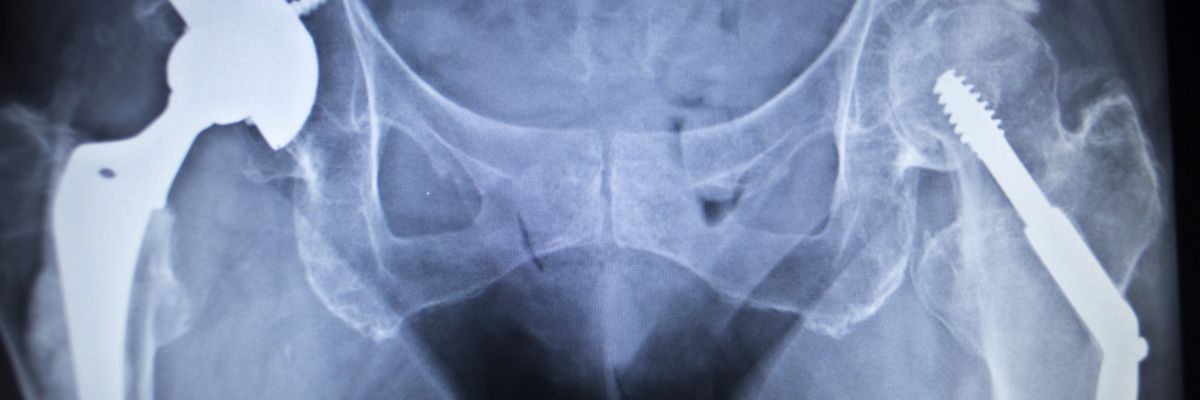
How to reinforce the human body with biodegradable metals
15. 01. 2025
In orthopedics, bones sometimes have to be stabilized using metal screws, plates, or wires, which are later surgically removed. But what if these materials could simply dissolve inside the body instead? Jan Pinc from the Institute of Physics of the CAS focuses on biodegradable metals that could be used for this very purpose. The article was first published in Czech in the A / Magazine of the CAS.
"Rust is boat cancer." / "I’m sorry. When I was a kid, I lost a bike to that." This line from the cult sitcom Friends serves as an apt introduction to the topic at hand. Corrosion can strike fear into the hearts of car owners, cyclists, and those with tin roof cottages alike. Metals exposed to harsh weather conditions quite literally fall apart. And the human body is an even more challenging environment – with fluids flow constantly, the body is relatively warm and teeming with ions and a variety of chemical compounds with differing affinities, charges, and reactivities.
It took years of research to develop the perfect metal compositions for medical use – metals that wouldn’t release harmful substances into the body and would remain safe for human use. Today, such implants are commonly utilized. However, having mastered alloys that can withstand the body’s demanding conditions, scientists and medical professionals are now pursuing the opposite goal: finding metallic materials that degrade within the body instead. The rationale? Practicality.
A CNC device used for manufacturing small orthopedic implants. (CC)
In some cases, doctors implant metal orthopedic components into the body only temporarily, which later have to be removed under general anesthesia – for instance, in cases of comminuted or complicated fractures. And what if the tissue not only needs to heal but also grow? In children, fixed metal implants can interfere with bone growth, potentially causing deformities. Here’s where biodegradable metals come into play – materials strong enough to stabilize a bone but capable of breaking down in the body over time, eliminating the need for a second surgery.
Absorbable screws, plates, and splints could also be used beyond orthopedics – biodegradable stents could find applications in cardiology, for instance. And the potential doesn’t stop there, given that 80% of all implants used in medicine are made of metals. Biodegradable materials could offer many advantages, but several obstacles still prevent their practical use. “Above all, they must be non-toxic and safe for the human body,” explains Jan Pinc from the Institute of Physics of the CAS, who is working on developing such materials.
Metals with issues
Magnesium, iron, and zinc appear to be the most promising candidates for biodegradable metals, but each has its drawbacks. Magnesium releases hydrogen gas as it degrades, leading to gas accumulation in the body (hydrogen molecules are very small). “It likely settles in fatty tissue, but no one knows for sure – it is still the subject of research,” Pinc says.
This isn’t magnesium’s only problem. It also degrades far too quickly. A standard orthopedic implant needs to last two to six months in the body, but magnesium breaks down well before that. At the other extreme is iron, which degrades far too slowly, and, under certain circumstances, its byproducts can harm the body.
Zinc currently seems to have the most favorable profile. It degrades at an ideal rate (about 0.3 millimeters per year), and its degradation products are non-toxic. On paper, zinc is a strong candidate, but it’s not without its issues. It falls short in terms of mechanical properties. To function, a component must retain sufficient strength even as it degrades to continue supporting, for example, a fractured bone.
This is where alloys come in. Zinc serves as the base material, with other metals added to it. The specific combinations and proportions of these additives need to be tested to determine what the resulting alloy can withstand and how it will behave in the body.
BIOREACTOR AND NEW ZEALAND RABBITS Testing on living organisms is mandatory for the approval of any material or implant. “As part of one project, we conducted tests on rabbit models. A special New Zealand breed must be used, and only males, so the results aren’t influenced by female hormones. The entire process is enormously expensive, and as soon as you slap a ‘scientific’ label on something, the price quadruples,” describes Jan Pinc, reflecting on the costs of purchasing mammals for testing. For ethical and practical reasons, he focused on developing his own bioreactor to at least partially replace in vivo testing. |
Testing, testing… and more testing
The development of biodegradable metals is still in its infancy and will require extensive research and testing. Factors such as temperature play a significant role. Laboratory tests performed under standard conditions can yield misleading results because they don’t replicate the body’s environment, which operates at 37°C. “For some metals, this doesn’t matter much. However, we’ve found that temperature significantly affects the mechanical properties of zinc,” explains Pinc, who focuses on testing potential future implants.
Conventional tests using cell cultures are designed for metals that are not meant to degrade in the body, such as titanium screws or joint replacements, and aren’t suitable for biodegradable metals. Before these materials can be used in practice, they must also undergo in vivo testing in living organisms – a process that is both time-consuming and expensive.
It would be advantageous if lab tests could replicate the conditions that implants will face in the body as closely as possible. “That is why my goal is to develop a bioreactor that simulates conditions like fluid flow, oxygen levels, and body temperature. These factors are usually neglected in lab tests – for example, corrosion testing is often done by simply immersing the material in liquid, which is quite different from the actual conditions the material will encounter in the human body,” Pinc explains.
Ing. JAN PINC, Ph.D. Jan Pinc studied biomaterials and metallurgy at the University of Chemistry and Technology in Prague (VŠCHT). He worked at the Czech Technical University (ČVUT) and since 2018 has been a researcher at the Institute of Physics of the CAS in the Department of Functional Materials. Pinc specializes in biodegradable zinc-based materials. His goal is to minimize animal testing, utilize AI in development, foster collaboration with international partners and domestic manufacturers of metallic implants, and promote interdisciplinary knowledge-sharing in the field of implantology. Pinc described the degradation mechanism of Zn-Mg alloys, which was published in Bioactive Materials. In 2024, he received the Otto Wichterle Award of the CAS for young talented scientists. |
To complicate matters further, the conditions in the body are not constant – they can vary. For instance, pH levels: these metals are stable at a pH of around seven. However, if the body is fighting inflammation – a common occurrence with injuries – the pH in the affected area drops. This introduces yet another variable that can influence how a biodegradable metal component behaves.
Today’s and tomorrow’s challenges
As we’ve seen, metals face a harsh environment inside the human body, and scientists face a tough challenge as well. Pure metals are practically unusable for these purposes due to the reasons already mentioned – there are simply too many drawbacks. Instead, scientists work with alloys. Essentially, another metal is incorporated into the base metal. This creates what are known as “phases” in the material – miniscule grains of another metal embedded in the matrix of the base metal – in this case, zinc. In one section, there might be more magnesium atoms than elsewhere. Different parts can even behave differently in the body, such as degrading at varying rates. It’s a bit like Swiss cheese – or a three-dimensional spider web.
To modify the material’s properties, processes like extrusion are used. In this process, the metal is forced through a kind of press, similar to a pasta maker. The resulting “spaghetti” has a more homogeneous structure than the original alloy. At the same time, this method can alter the material’s mechanical properties. The processing reduces the size of the grains and arranges them in a specific orientation within the structure. As a result, the material can have mechanical properties and exhibit different strengths depending on whether pressure is applied from below or from the side. “This is a very advantageous property, for example, when using an orthopedic plate in the leg, where forces act on the component to varied degrees in each direction,” Pinc adds.
A damaged screw analyzed using X-ray microtomography. (CC)
The properties of the material – such as its degradation rate – can also be adjusted using special coatings. Researchers at the Institute of Physics of the CAS are planning to work with colleagues from the Institute of Rock Structure and Mechanics of the CAS and the Department of Materials at the Faculty of Nuclear Sciences and Physical Engineering of the Czech Technical University in Prague to coat components with specific polymer layers. They are also planning to launch a European project in collaboration with researchers from France in 2025.
For specialized applications, the surface of the metal can even be bombarded with nitrogen ions, which integrate into the material’s surface. This creates enormous internal pressures and forms tiny pores in areas where the nitrogen impacts. “We are capable of creating highly porous, nanometer-thick layers that are also uniform, and we’re considering their use as drug carriers,” Pinc explains. This is still a preliminary idea that he hopes to develop with colleagues from the Czech Technical University and Slovakia.
There are even ideas involving 3D printing. And that’s without mentioning the sterilization of these components, which is no simple feat either. It’s fair to say that scientists face more than enough challenges ahead in this field.
Future prospects
Jan Pinc now has access to a new powder metallurgy laboratory, where precise mixtures of metallic powders can be prepared for the desired alloys. The material is created through sintering, using extremely high currents. One advantage of this process is that the resulting material can be relatively porous. It can even be used to create lightweight “scaffolds” for additional fillings. However, every version of the material must not only fulfill its intended purpose but also function as an integrated whole.
TWENTY-FIRST-CENTURY WIREWORKING At the Institute of Physics of the CAS, researchers can produce special wires made from biodegradable alloys, with a thickness of just 300 microns. They are currently working on coating these wires with polymer layers. Such wires could then be used in specific applications. “For instance, if you suffer a complicated fracture in the middle joint of your finger, two holes are drilled, and the bones are tied together with wire. Similarly, during heart surgeries, the opened rib cage is later tied back together with wire,” Pinc explains. |
Scientists at the Institute of Physics of the CAS are tackling the problem holistically, studying not just isolated traits of the material but designing entire functional systems that would work effectively in the body. For instance, they have developed an orthopedic plate – a small flat piece with special screws.
“We want to test it in terms of anisotropic mechanical properties – that is, how it handles mechanical stress from different directions. And we don’t just want to test each part individually but as an integrated system. In connection with this, it’s also necessary to determine the degradation behavior under the same conditions because this brings up a host of additional issues,” Pinc explains.
One of these issues could be the connection between the plate and the screw head. A tiny gap – no more than 10 µm – will inevitably form between them, where the exchange of ions with the surrounding environment will be limited. In such areas, the local environment could become more aggressive. This might lead to the screw head degrading too quickly and falling off. Naturally, the entire plate system would then become nonfunctional.
RUST IN THE BODY Metals inside the body don’t rust like iron railings outside on the street. Instead, they break down – or more accurately, dissolve. At points of contact with albumin, amino acids, or inorganic compounds, the metal begins to dissolve into the surrounding environment as ions. “These ions interact with others, and a very thin layer of phosphates starts to form on the surface. For zinc, at least, these aren’t harmful to the body. This creates a kind of barrier that slightly reduces the corrosion rate. In most cases, much more complex compounds form. What’s crucial is that they aren’t toxic to the body,” Pinc explains. |
The screw itself also poses a challenge. With a titanium screw, the surgeon can apply significant force. Zinc, however, is more brittle and could break. The material must therefore be designed to withstand the necessary handling.
This entire issue requires an interdisciplinary and comprehensive approach to problem-solving. Experts in metals, other materials (polymers, ceramics), chemists, biologists, and medical professionals are all needed. But one day, we may very well see biodegradable metallic zinc-based implants used in the human body for orthopedic applications. And for that purpose, we might finally make peace with rust.
“With advances in artificial intelligence, I believe we’ll reach a point where the material selection process, which we currently base on our experience, will be significantly accelerated. Then we’ll be able to predict the properties of individual alloys or materials – or even the components themselves – before we manufacture them,” Pinc envisions, optimistic about the future.
*
The article was published in Czech in the CAS quarterly A / Magazine, 4/2024 (version for download):
Written by: Viktor Černoch, External Relations Division, CAO of the CAS
Translated and prepared by: Tereza Novická, External Relations Division, CAO of the CAS
Photo: Jana Plavec, External Relations Division, CAO of the CAS; Shutterstock
The text and photos labeled CC are released for use under a Creative Commons license.
Read also
- Renewables are a strategic investment in European security, scientists say
- New record set for neutrino mass: one million times less than an electron
- On the trail of the endangered little owl: searching for newly hatched owlets
- Teen scientist breaks barriers: No child is a lost cause
- The Czech Academy of Sciences has appointed its new leadership for 2025–2029
- Literature is tied to its historical context. How can we read between the lines?
- Radomír Pánek: The Academy must be united, strong, and proactive
- A springtime booster: The healing potential of tree buds
- A novel compound that protects bone cells may benefit diabetic patients
- New interactive exhibition at the CAS showcases materials shaped by genius ideas
The Czech Academy of Sciences (the CAS)
The mission of the CAS
The primary mission of the CAS is to conduct research in a broad spectrum of natural, technical and social sciences as well as humanities. This research aims to advance progress of scientific knowledge at the international level, considering, however, the specific needs of the Czech society and the national culture.
President of the CAS
Prof. Eva Zažímalová has started her second term of office in May 2021. She is a respected scientist, and a Professor of Plant Anatomy and Physiology.
She is also a part of GCSA of the EU.